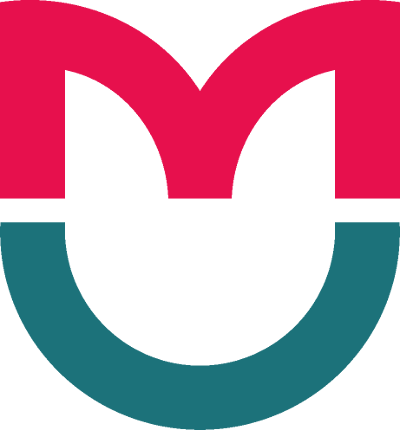
METHOD
Gadolinium- and curcumin-loaded micelles based on α-fetoprotein functionalized amphiphilic block copolymers
1 Laboratory of Radionuclide and Beam Technologies in Experimental Oncology,N. N. Blokhin Russian Cancer Research Center, Moscow, Russia
2 Laboratory of Light Sensitization,N. M. Emanuel Institute of Biochemical Physics, RAS, Moscow, Russia
Correspondence should be addressed: Natalia V. Pozdnyakova
Kashirskoe shosse, d. 24, Moscow, Russia, 115478; ur.liam@2002optan
Funding: the study was supported by the Ministry of Education and Science of the Russian Federation (Grant Agreement no.14.607.21.0133 dated October 27, 2015, Project ID RFMEFI60715X0133).
Due to its unique paramagnetic properties, gadolinium (Gd3+) holds a firm place in medical diagnostics (magnetic resonance imaging, MRI) and therapy (secondary thermal radiation used for eliminating tumor cells) [1]. Electron spin-lattice relaxation time T1 of gadolinium-based contrast agents is about 0.1 ns, i. e., an order of magnitude shorter than proton relaxation time in water [2]. Since Gd3+ is very toxic even at low concentrations that are routinely used for enhanced MRI, it must be chelated with agents that have a high effective association constant, such as diethylenetriaminepentaacetic acid [3]. Nanoparticles containing Gd3+ chelates are widely used in clinical practice for performing contrast-enhanced MRI of various tissues on living patients, including scans of brain structures [4], vascular plexuses [5], myocardium and coronary arteries [6].
Kang et al. [7] have described a method of preparing gadolinium oxide nanoparticles Gd@SiO2-DO3A and Gd@SiO2-DO2A-BTA of 50–60 nm in size. Nanoparticles were synthesized from tetraethyl orthosilicate (TEOS) and (3-aminopropyl) triethoxysilane (APTES); then, aminopropylsilane groups were functionalized with 1,4,7,10-tetraazacyclododecane-1,4,7,10- tetraacetic acid (DOTA) or 1,4,7,10-tetraazacyclododecane-1,4,7-triacetic acid conjugates of benzothiazole (DO3A-BTA). The obtained nanoparticles were highly soluble in water; their colloid solution was stable. Their relaxivity r1 was much higher than r1 of low molecular weight contrast agents; the ratio r2/r1 [8] was close to 1, which indicates that the investigated nanoparticles can be used as MRI contrast agents [9]. Biodistribution analysis showed that Gd@SiO2-DO2A-BTA is excreted in bile and urine. The nanoparticles also accumulated in tumor cells and were found efficient for in vivo elimination of such continuous cell lines as SK-HEP-1, MDA-MB-231, HeLa and Hep 3B. Although some authors report cumulative toxic effects of nanostructured gadolinium-based contrast agents [10, 11], including renal toxicity [12], the latter remain an attractive subject for research, as they can be used in MRI and cancer therapies [13].
The aim of this work was to elaborate a technique for obtaining a Gd3+ — based low-toxic micellar formulation for visualizing tumor tissues in vivo.
METHODS
To ensure that the system is low toxic and has affinity to tumor cells and to prevent its non-specific binding to normal tissues, three solutions were suggested.
First, we decided to use curcumin as a complexing agent. Curcumin is a non-toxic natural compound (fig. 1). It is abundant in the roots of Indian saffron Curcuma longa. Some of its properties come in handy for our research [14]: it can form stable coordinate bonds with transition metals [15], it is not toxic at usual concentrations, and its hydrophobicity allows accommodating its compounds in the micelle core and ensures micelle stability in aqueous solutions.
Second, we obtained a micellar formulation of the compound. Methods of preparing micellar formulations have already been employed by biotechnology [16] and can possibly find their way into pharmaceutical industry [17]. Micellar formulations have some advantages over other types of carriers: in aqueous solutions, they self-assemble into structures with a hydrophobic core and a hydrophilic surface, which makes it possible to accommodate hydrophobic agents inside the micelle and thus protect them from inactivation in biological media. Micelles are small (<100 nm), easy to formulate and have long blood circulation time [18]. Among the advantages of block copolymer (Pluronic)-based micelles are low toxicity, weak immunogenicity and simplicity of surface modification with functional groups necessary for obtaining certain properties. For this work, we chose amphiphilic triblock copolymers of polyethylene glycol and polypropylene glycol Pluronic F-127 and Pluronic P-123 (Sigma-Aldrich, USA) (fig. 2)
Third, we improved the technique of conferring target specificity to a micelle by immobilizing to its surface a recombinant vector protein (a fragment of human alpha- fetoprotein with high affinity to tumors of various types) [19].
The following reagents by Sigma-Aldrich, USA, were used in the study: gadolinium(III)nitrate hexahydrate, triblock copolymers Pluronic F-127 and Pluronic P-123, triethylamine, curcumin, 1,1′-carbonyldiimidazole, N-hydroxysuccinimide, 2,4,6-trinitrobenzenesulfonic acid, tetrahydrofuran, dimethyl sulfoxide, methanol, dimethylformamide, ethanolamine, cobalt thiocyanate, barium hydroxide. The protocol for obtaining human alpha-fetoprotein-based vector protein was described previously [19].
Preparation of curcumin–gadolinium complexes
Complexes were prepared in the organic solvent tetrahydrofuran by mixing gadolinium nitrate, curcumin and triethylamine at molar ratio of 3:1:1, heating the mixture to 50 °C for 30 minutes. Then, triethylamine and the solvent were removed in the rotary evaporator. Details are given below. 100 μl of 0.5 M curcumin solution in tetrahydrofuran were mixed with 300 μl of 0.5 M gadolinium nitrate solution in tetrahydrofuran. Then, 100 μl of 0.5 M tryethylamine solution in methanol were added dropwise over 1–2 minutes while stirring the mixture vigorously. The mixture was heated to 50 °C for 30 minutes while stirring. Solvents and triethylamine were removed from the solution at 50 °C using the rotary evaporator; the solids were washed in methanol and air-dried.
Qualitative assessment of Gd3+ complex formulation was performed on the Cary 50 Scan UV-Vis spectrophotometer (Agilent Technologies, USA) with a scan range set to 300– 650 nm, in a 1 cm-pathlength disposable cuvette (Sigma- Aldrich, item no. Z330418). Dry aliquots (weight ranging from 1 to several mg) were collected with a spatula. Mother liquor was diluted down with dimethyl sulfoxide (DMSO) to obtain the optical density of 0.5 to 1.5 at 450 nm. To determine complex concentration, the peak height was measured at 455 nm (with free curcumin the peak is absent). To find the peak height [20], we deducted spectral data obtained from free curcumin solution in DMSO from the spectral data of the reaction mix.
Preparation of a modified copolymer Pluronic F-127 with NHS end groups for vector protein immobilization to micelle surface
Pluronic F-127 was modified in dimethylformamide (DMFA) in two steps. At first, hydroxyl end groups were activated by carbonyldiimidazole (CDlz). Next, a reaction with N- hydroxysuccinimide (NHS) was carried out (fig. 3). Briefly, 204 mg of Pluronic F-127, 19 mg of NHS and 26 mg of CDlz were dry-mixed. Then, DMFA was added to bring the final volume of the reaction mixture to 500 μl. The mixture was stirred for 1 hour at 37 °C until CO2 gas bubbling ceased. Excess CDlz was removed by adding 50 μl water to the reaction mixture. After excess CDlz was hydrolyzed, the modified copolymer was extracted several times with diethyl ether. The solids were air-dried.
The number of NHS end groups in the modified Pluronic F-127 was measured by performing a reaction with excess ethanolamine in 10 mM borate buffer (pH 8.5) with subsequent titration of unreacted amino groups by 2,4,6-trinitrobenzenesulphonic acid (TNBS), as described previously [21].
Micelle formulation
Various copolymer-based micellar systems were prepared as described below (fig. 4). Weighted amounts of the curcumin/ gadolinium complex and modified pluronics were dissolved in tetrahydrofuran and mixed together. The amount and the ratio of the components were different in various micellar systems (see the table). Then, the solution was poured into a rotary evaporator flask, and the organic solvent was removed at 50 °C while the flask was rotating. Then, distilled water was added and the mixture was stirred vigorously for 15 minutes and centrifuged. Supernatant was removed; the mixture was dialyzed against the phosphate-buffered saline (pH 7.5) at +4 °C for 24 hours.
To assess how efficiently the investigated substance was encapsulated into the micelles (%), the following formula was applied, where Menc is the mass of the encapsulated curcumin-gadolinium complex, and Minit is the initial mass of the curcumin-gadolinium complex. Concentration load (% m/m) was calculated according to the following formula, where Mpol is the copolymer mass.
Chemical modification of micelle surface
Micelles were conjugated to protein by mixing the copolymer-based micellar solution and the protein solution, in molar excess of the modified copolymer (NHS groups) to the protein. For functionalization, we used recombinant domain 3 of human alpha-fetoprotein [19]. The detailed protocol is below. 1 ml of the micellar solution was mixed with 200 μl of 0.1 mM protein solution (concentration of 3 mg/ml) in the phosphate-buffered saline (pH 8.0) and incubated for 1 hour at room temperature. To deactivate excess reactive NHS groups, excess ethanolamine was added after incubation, until its concentration in the solution was 10 mM; the mixture was then incubated at room temperature for 2 hours and dialyzed against the phosphate-buffered saline (pH 7.2) at +4 °C for 24 hours. The obtained substance was stored at +4 °C for one week.
Qualitative detection of protein in the obtained micelle composition was performed in a polyacrylamide gel by electrophoresis with subsequent staining for 10 minutes in a 5% aqueous solution of barium iodide [22].
Evaluation of micelle size
The average size of the micelles and size distribution were evaluated by dynamic light scattering on ZetasizerNano ZS (Malvern Ltd., UK) in thermostatic plastic microcuvettes ZEN0040. Measurements were performed using the helium- neon laser with a wavelength of 633 nm and a power level of 4 mW at 25 °C. Prior to measurements, samples were diluted tenfold with deionized water. Measurements for each sample were performed in replicates of 3. Mean root square deviation was calculated.
Determination of gadolinium content in micelles
Measurements were performed on the X-ray fluorescence analyzer X-art M (Komita, Russia). The signal from the studied samples was compared to the signal from the solutions of known gadolinium concentrations.
Determination of curcumin content in micelles
To determine curcumin content, absorption spectra of the micelles and calibration solutions of known concentration were measured in a disposable cuvette (Sigma-Aldrich, item no. Z330418) at 430 nm wavelength on the Cary 50 Scan UV-Vis spectrophotometer.
Measuring polymer dry matter content
Dry matter content was measured using a previously described method [23] with slight modifications. The reagent was prepared as follows: 0.2 ml of 2.6 M aqueous solution of cobalt thiocyanate (CoSCN) were mixed with 0.8 mL of 0.8 M barium hydroxide aqueous solution. A 10 μl aliquot of pluronic- containing solution was mixed with 10 μl of the reagent, stirred vigorously and centrifuged; supernatant was removed; the solids were air-dried. Then, 100 μl of DMSO and 5 μl of 5 M HCl were added to the solids. Absorption was measured at 630 nm on the Titertek Multiscan Plus reader (LabX, Canada) in 96-well plates. Calibration solutions of known concentration were measured in parallel. Using a calibration curve, concentration in the analyzed sample was calculated.
Determination of total protein content
Total protein content was evaluated by a modified Lowry method using bicinchoninic acid (Sigma-Aldrich). A standard bovine serum albumin solution was used as a calibration solution [24].
Assesment of toxicity of curcumin-gadolinium micelle formulation
To verify that further in vivo research is possible, we investigated animal tolerance to the obtained micelle formulation. We used 10 C57/black female mice with an average weight of 25 g. The animals were administered the micellar formulation composed of 10 mM Gd3+, 10 mM curcumin, 5 mM Pluronic F-127 and phosphate-buffered saline (pH 7.0). Micelle diameter was 20 nm. The animals received a single 200 μl injection into the tail vein. Dosage was selected based on the pluronic concentration optimal for delivering the cytostatic drug Docetaxel to lung tumor cells in vivo in the mice model [25]. The animals were observed for 2 months. Then, they were sacrificed; autopsy and histological analysis were performed subsequently.
RESULTS
The process of curcumin-gadolinium complex formation was controlled by recording spectral changes, such as the additional peak occurring at 455 nm [20] (fig. 5).
The copolymer:NHS ratio in the modified pluronic F-127 was about 1:1. In total, we obtained about 100 μl of dry modified copolymer containing one NHS group per molecule.
Encapsulation efficiency of the method applied for micelle formulation varied from 40 to 80 % and load concentration varied from 10 to 25 % m/m. As a result, we obtained a number of micelle solutions with various amounts of basic components (see the table).
Curcumin/gadolinium ratio in the micelles implies vacant valence orbitals in a gadolinium atom that are presumably occupied by water molecules, which is essential for preserving contrasting properties during MRI scans [26]. As far as micelle stability goes, we should note that evaluation of micelle sizes by dynamic light scattering and measurements of visible absorption spectrum typical for the curcumin-gadolinium complex were repeated after the micelles had been stored at +4 °C for 10 days. All values remained unchanged. Differences fell within the standard error of measurement.
The size of the obtained micelles was approximating (but no exceeding) 100 nm. It allows micelles to circulate in the blood for a long time without being captured by the hepatic portal and reticuloendothelial systems [27]. This property is important for ensuring that micelles are delivered to tumor tissues, owing to the immobilized functional protein agent on their surface [28].
During the experiment on mice, no visible signs of toxicity of the micelle system were detected. We did not observe symptoms of heavy metal poisoning [1], such as collaptoid reactions or bleeding. The animals had a good appetite and were active; there were no signs of pathological thirst or photophobicity.
DISCUSSION
A number of works [14, 29] prove that curcumin can be used as a complexing agent that suppresses gadolinium toxicity in vivo. Patil et al. [29] used such complexes for detecting amyloid plaques in Alzheimer’s patients. The researchers exploited the natural affinity of curcumin to β-amyloids. Our work is the first to demonstrate the possibility of encapsulating hydrophobic curcumin complexes into micelles formed by pluronics (block copolymers of polyethylene glycol and polypropylene glycol). Such micelles exhibit stability in aqueous media, therefore, curcumin pharmacokinetic properties no longer play a key role in distributing Gd3+ ions in body tissues, because the chelated complex remains highly stable.
Other authors [7] worked on an almost identical task, but found a different solution to it: they encapsulated Gd3+ ions into silicon. It proves that the task itself is of high practical significance. The technique we suggest has an advantage over the one described in work [7]: it can be implemented in a biochemical laboratory using commercially available reagents and standard equipment, with almost 100 % yield and without recruiting professional synthetic chemists. This technique also allows both small and commercial scale production of Gd3+ containing nanoparticles. Despite its simplicity, the absolute size of particles, size distribution and the amount of the encapsulated substance do not differ from the corresponding values suggested in [8]. It became possible due to those curcumin properties that make it a natural Gd3+ chelator.
Micelles have an important advantage over hard silicon oxide nanoparticles. They can circulate in the blood for a long time without being destroyed by liver monocyte derivatives [9]. At the same time, defective or leaky tumor vasculature allows micelles to be retained in tumor tissues [30].
Additional enhancement of target specificity of Gd3+ containing micelles can be achieved through using various target-specific functional groups. Domain 3 of α-fetoprotein employed for this work has a high affinity to receptors of various tumor types, since, like albumin, it uses them as a nutrient [19]. The suggested technique can efficiently immobilize any protein, peptide or other target-specific groups to micelle surfaces.
CONCLUSIONS
We have demonstrated that curcumin-gadolinium complexes are efficiently encapsulated and strongly retained in micelles formed by polyethylene glycol and polypropylene glycol copolymers (pluronics). Stored at +4 °C for 10 days, the micelles exhibited no changes in their properties that would not fall within the standard error of measurement. The micelles have a standard size of 50-100 nm depending on the ratio of their components and a prolonged blood circulation time.
We suggest a technique for modifying the surface of pluronic mycelles containing curcumin and Gd3+ complexes using target-specific molecules, such as domain 3 of human α-fetoprotein.
Due to its simplicity, high efficiency and a possibility to work with scant amounts of components, this method is intended primarily for studying distribution of macromolecules and their complexes in vivo. It can also be used for screening functional target-specific groups that deliver biomacromolecules and their complexes to tissues and cells of various types, including tumors.