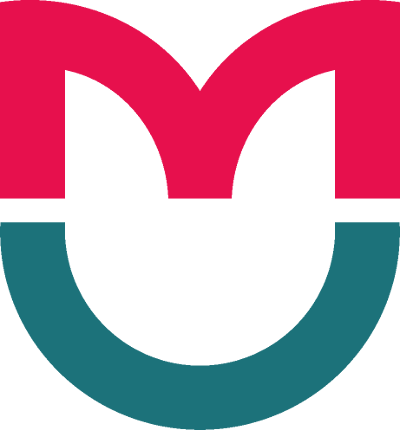
ORIGINAL RESEARCH
Interictal epileptiform activity in sleep and wakefulness in patients with temporal lobe epilepsy
Research Center of Neurology, Moscow, Russia
Correspondence should be addressed: Amayak G. Broutian
Leningradsky Prospect, 33, Moscow, 125284; ur.liam@naituorba
Author contribution: Broutian AG — research planning, literature analysis, data interpretation, manuscript drafting; Belyakova-Bodina AI, Abramova AA — data collection, analysis, interpretation, manuscript drafting; Dolgova SM, Pushkar TN — data collection, analysis.
The likelihood of developing epileptic seizures depending on the level of wakefulness and sleep depth is largely determined by the epilepsy and seizure type [1, 2]. For example, in juvenile myoclonic epilepsy seizures typically occur after awakening while in autosomal dominant nocturnal frontal lobe epilepsy they only happen during sleep. Sleep is also known to be a significant activator of interictal epileptiform activity in electroencephalography (EEG), which is more common for focal discharges than for generalized ones [3].
Sleep, however, is not a homogeneous state; it consists of several states changing in a cyclic manner. These states differ in depth, physiological parameters, and EEG patterns. A remarkable example is epileptic encephalopathy with continuous spikes and waves during sleep (CSWS) in which epileptic discharges almost replace the physiological patterns of non-rapid eye movements sleep (NREM sleep) and disappear during rapid eye movements (REM) sleep.
Thus, the probability of epileptiform activity is expected to depend on sleep stage. Furthermore, ED number during a certain sleep stage should be related to the duration of this sleep stage. For example, stage 2 prevails in a healthy person’s sleep, which may mislead an EEG interpreter into thinking that EDs are the most prevalent during this stage. Epileptiform discharge index (EDI) is described for both generalized and focal forms of epilepsy [:lit_4–8]. Studies on focal discharges differ in number of patients, discharge localization and epileptiform activity index calculation methods. In the majority of studies, discharges were calculated in 5 to 20-minute intervals of wakefulness and each stage of sleep. Both discharge localization and calculation methods can affect the results [4–8].
Temporal epilepsy is the most common, comprising two thirds of all focal epilepsies [9]. The aim of this study was to assess the effect of various stages of sleep on interictal epileptiform activity in a relatively homogeneous group of adults with temporal lobe epilepsy by counting and analyzing EDs throughout the entire EEG recordings.
METHODS
We scanned video EEG recordings obtained in the Research Center of Neurology from February 2018 through June 2019 to select overnight recordings only. We found 709 recordings which were 10 to 12 hours long and included night sleep, evening and morning wakefulness. Initially, 142 recordings with focal epileptiform activity regardless of the disease duration and antiepileptic drug regimen were chosen. Inclusion criteria were the following: 1) only temporal ED localisation; 2) in case of repeated recordings, the first one was analyzed; 3) all sleep stages were present; 4) manual ED detection only, no automatic algorithms were used; 5) at least 10 EDs in a recording. Exclusion criteria were: 1) epileptic seizures during recording; 2) more than 500 EDs. Recordings with epileptic seizures were excluded due to their potential effect on EDI. Recordings with more than 500 EDs were excluded because of the technical limitations of ED manual detection. Finally,
32 recordings that met all the criteria were included in our study. Among the included patients there were 17 females and 15 males, aged 19 to 79 years (median 41 years).
EEG was recorded with scalp electrodes placed according to 10–20 international system with additional EKG channel. In 11 recordings, an inferior temporal chain (F9, F10, T9, T10, P9, P10) was used as well. EEG was recorded with NicOne (Natus, USA) and BePlus LTM (EBNeuro; Italy) systems. Recordings started between 20:00 and 21:00 and ended between 07:00 and 08:00. Photic stimulation and 5-minute hyperventilation were performed in the beginning of the recordings and after awakening in the morning. Epileptiform activity was defined according to 2017 revised glossary of EEG terms [10]. Total number of EDs varied from 13 to 401 (median 72 EDs). 27 patients (84%) showed unilateral EDs, 5 patients showed bilateral EDs. Number of patients with left-sided or predominantly left-sided EDs (17) was comparable to the number of patients (15) with right-sided or predominantly right-sided EDs.
Sleep was staged using 30-second epochs in accordance with American Academy of Sleep Medicine Manual for the Scoring of Sleep and Associated Events [11]. Since we did not use electrooculographic (EOG) electrodes and electromyographic (EMG) chin electrodes, REM sleep was identified based on indirect signs, such as ocular artefacts in frontal channels, muscle artifacts and REM-sleep-specific EEG patterns [11]. In this paper, we used traditional designations for sleep stages and wakefulness: Wake for wakefulness, N1 for stage 1, N2 for stage 2, N3 for stage 3 (slow-wave sleep), REM for REM sleep. fig. 1 shows an example of a hypnogram with ED marks.
We created hypnograms and marked EDs on them for each recording and calculated the following indices: 1) absolute general EDI, defined as a ratio of total ED count to the full recording time in hours; 2) absolute EDI for wakefulness and each sleep stage (N1, N2, N3, REM), defined as a ratio of ED count during stage to its duration in hours. For bilateral EDs, average EDI from both sides were calculated. The range of absolute EDIs was substantial. Since our study mainly focused on the trends of EDIs in relation to sleep stages and wakefulness, we did additional normalization, calculating relative EDIs, defined as a ratio of absolute stage EDI to absolute general EDI. Further in the text, EDI should be regarded as relative EDI, unless otherwise specified.
STATISTICA 12.6 software (Statsoft, Dell Software; USA) was used for statistical processing of the results. We applied the Shapiro–Wilk normality test for distribution characteristics, Mann–Whitney test for independent samples and Wilcoxon test for dependent samples. Spearman's rank correlation coefficient was used to calculate the correlation. The differences were considered statistically significant at p < 0.05.
RESULTS
18 patients (56%) had EDs while being awake, 16 patients (50%) had EDs in N1, 31 patients (97%) had EDs in N2, all the patients had EDs in N3 and 9 patients (28%) had EDs in REM sleep (table). The combinations of wakefulness and sleep stages with EDs varied (fig. 2). No recording showed EDs exclusively in wakefulness, N1, N2 or REM. Nevertheless, one recording revealed EDs only in N3.
Stages with the largest EDI were identified. 28 patients (87.5%) had the largest EDI in N3, 2 patients had the largest EDI in wakefulness period, 1 patient had the largest EDI in N2, 1 patient had the largest EDI in REM, no patients were shown to have the largest EDI in N1.
Relative EDIs with trends are shown in fig. 3. The median EDIs were: 0.03 for wakefulness periods, 0.11 for N1, 0.19 for N2, 3.92 for N3, 0.19 for REM (table, fig. 4). Application of the Wilcoxon signed-rank test revealed a significantly higher EDI in NREM sleep compared to that of wakefulness: p < 0.05 for N1 and p < 0.01 for N2 and N3, with difference from REM being not significant. N3 EDI was significantly larger than EDI for other stages. EDI for REM was significantly smaller than EDI for each NREM sleep stage. EDI for REM had no significant difference from EDI for wakefulness period.
We divided our sample into 2 groups according to the total ED number: patients with small ED number (14 to 75 EDs) and large ED number (75 to 401 EDs). We compared EDIs between these groups and found no significant difference.
We performed correlation analysis for absolute stage EDIs and found significant correlation between EDI for N1 and REM, N1 and N2, and N2 and N3.
There was no significant EDI difference between left and right side temporal discharges in either wakefulness or sleep.
DISCUSSION
Our study showed N3 EDIs to be substantially higher than EDIs in other stages. ED predominance in slow-wave sleep was shown in earlier studies, in which EDI was analyzed for each sleep stage [6–8, 12–14]. There were no patients without EDs in N3 in our study, and EDs in N3 were on average three times more probable than in N2. Comparing stages that harbored the majority of EDs within a single EEG recording revealed even greater difference, as recordings with the majority of EDs in N3 had a share of 87.5%. Analyzing the numerical data (table) and EDI trending (fig. 3), we observed a burst in N3. Similar to N3, EDI in N2, being smaller than in N3, was still significantly larger than in wakefulness, N1 and REM. We found no previous studies that showed significant difference in EDIs for N1 and N2. The lack of such statistical significance in earlier studies may be attributed to the method of ED marking, which was performed in selected intervals of EEG recordings rather than throughout the whole recordings [8, 15, 16].
Hence, we observed EDI increasing with sleep progression from REM to N1, N2 and N3.
Such EDI changes through the sleep stages may be explained by increased synchronization of neural networks in NREM sleep. Scalp EEG electrodes are known to require quite a large number of cortical neurons to discharge synchronously. Experimental data suggest that such synchronously discharging neurons should occupy at least an area of 6–8 cm2. Slow activity, mainly that of delta range, is known to reflect high synchronization of cortical neurons. A characteristic feature of N3 is high-amplitude delta activity occupying at least 20% of an epoch. However, thorough analysis may reveal gradual increase of slow-wave activity occurring already in N2. Apart from slow-wave activity not sufficient to meet N3 criteria, N2 is characterized by the presence of K-complexes which is the most important criterion of this sleep stage. K-complexes are relatively slow high-amplitude transients which may embed EDs, becoming the so-called epileptic K-complexes. Such epileptic K-complexes can be found in both generalized and focal epilepsy [17, 18]. Furthermore, nocturnal frontal lobe epilepsy seizures have been shown to be associated with K-complexes [19]. Hence, an increased probability of EDs in NREM sleep may be considered as a consequence of increased synchronization of neural networks. This is supported by studies which showed positive correlation between ED frequency and delta band power.
Despite sleep in general being the most potent ED activator, REM sleep harbored the least of sleep EDs, with the index of epileptiform activity being comparable to such of wakefulness. Our data are consistent with earlier studies of the effect of REM sleep on epileptiform activity [8, 14, 21, 22]. In all the studies, EDI in REM was the lowest among sleep stages. Compared to wakefulness, EDI in REM sleep was shown to be slightly lower in the majority of studies. Having no activating effect on epileptiform activity, REM sleep, however, has two aspects that draw our attention. Firstly, if occurring in REM sleep, EDs tend to be more localized, thus having greater localizing value than in other sleep stages [23, 24]. Secondly, REM sleep, being an integral part of healthy sleep, not only has no activating effect on epileptiform activity, but also reduces its probability. Thus, to some extent, activity of neural networks in REM sleep is protective, reducing the probability of not only EDs, but also seizures [3].
Our study results might have been affected by several factors. Firstly, we intentionally excluded patients with exceedingly large numbers of EDs, which were hard to be counted manually. In some earlier studies, ED counting was selective, i.e. EDs were marked in limited stretches of time (5–20 minutes to 1 hour) with further extrapolation to the entire EEG recording [8, 14, 15, 25]. We also occasionally use selective ED count in practice; however, we did not include recordings analyzed in such a way in order to avoid probable bias. Secondly, REM sleep duration might have been underestimated because of the lack of EMG channels reflecting the activity of mentalis muscle. Thirdly, wakefulness EDIs might have been overestimated, as 5-minute hyperventilation tests included in wakefulness periods increase the neuronal synchronization by reducing cerebral blood flow and thus may activate ED. Nevertheless, NREM sleep is a much stronger activator compared to hyperventilation [15]. Fourthly, the effect of antiepileptic therapy, time from the first seizure, seizure frequency and semiology, drug resistance and structural lesions on MRI was not analyzed.
From a practical point of view, our study highlights the importance of slow-wave sleep recording in EEG diagnostic investigations, especially if focal epilepsy is suspected. Short-term EEG, particularly 20-minute routine EEG, provide lower probability to record EDs, thus diminishing sensitivity of these tests in the diagnosis of epilepsy, especially its focal forms. As a result, a precise diagnosis and correct treatment initiation might be delayed.
CONCLUSIONS
Our study on a sample of patients with temporal epileptiform activity showed that relative EDI for focal discharges increases while NREM progresses from N1 to N3. In REM sleep, EDI drops significantly to the level comparable to wakefulness. The most likely mechanism behind the significant predominance of EDs in the slow-wave sleep is synchronization of cortical neurons. Lack of records describing the N3 stage, which is when the epileptiform activity reaches its maximum, or the insufficient duration of this stage significantly reduce the likelihood of epileptiform activity recording, and when the clinical manifestation is insufficiently convincing, this lack of information can lead to erroneous conclusions, and to a delay in making the correct diagnosis and proper treatment initiation.