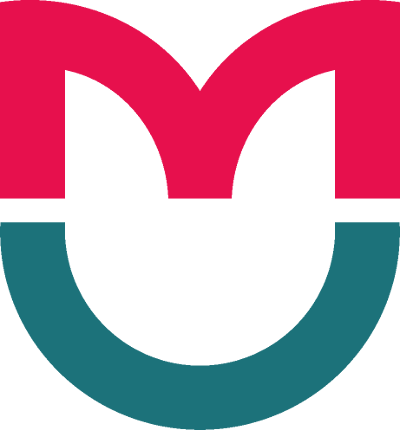
ORIGINAL RESEARCH
Biochemical and morphological substantiation of the connective tissue hypothesis of mandibular third molar eruption
1 Omsk State Medical University, Omsk, Russia
2 Central Research Institute of Dentistry and Maxillofacial Surgery, Moscow, Russia
3 Omsk State Pedagogical University, Omsk, Russia
4 City Clinical Dental Polyclinic № 1, Omsk, Russia
Correspondence should be addressed: Andrey S. Korshunov
Kosareva, 34, Omsk, 644043, Russia; ur.liam@885081_k_yerdna
Author contribution: Korshunov AS — study planning, literature analysis, data interpretation, clinical data acquisition, manuscript writing; Vagner VD — study planning, literature analysis, data interpretation; Belskaya LV — study planning, biochemical investigations, manuscript writing; Kuryatnikov KN — clinical data acquisition, literature analysis, data interpretation, manuscript writing; Serov DO — clinical data acquisition, data interpretation, literature analysis; Krasnov VA — clinical data acquisition, sample preparation for assessment; Tigranyan GO, Bondar IA — sample preparation for assessment, data analysis.
Compliance with ethical standards: the study was approved by the Ethics Committee of the Omsk State Medical University (extract from the protocol № 113 of 26 November 2019); the informed consent was submitted by all study participants or their legal representatives.
Tooth eruption is a unique natural process with multifactorial reproducibility mechanism that makes it possible to judge about the development of not only oral, but also the whole body’s organs and tissues [1]. Eruption is a complex biological event involving dynamic changes at the tissue and cellular levels [2]. It is guided by anatomical structures, biological and molecular factors that make the tooth move to its final functional position in the oral cavity [3].
During eruption the teeth move in three directions and gradually increase in size within the alveolar part of the mandible before active eruption. The tooth demonstrates minor circular movements when the coronal part is formed [4, 5].
Some tooth eruption theories were proposed centuries ago, many of these theories were revised, amended or disproved [6]. The best known and well substantiated ones are as follows: the tooth theory (Hunter, 1870), alveolar theory (L.J. Baume, 1890), pulp theory (G.V. Yasvoin, 1929), bone tissue rearrangement theory (J. Tandler, 1928; A.Ya. Katz, 1940; B. Orban, 1953; J. Reichborn-Kjennerud, 1959; М.Ya. Berry, 1968). Many authors accept the theory that the connective tissue embracing the erupting mandibular third molar is the most probable source of eruptive forces [7]. According to other data, the coronal and basal cells of tooth enamel and tooth follicle can send a signal that induces differentiation of the relatively nearby mesenchymal or progenitor cells, transforms these into cells having specific functions, i.e. osteoblasts and osteoclasts [8].
According to another hypothesis, vascular and tissue pressure can affect the eruption rate. The experiment involving dental alveoli of rats has shown that pressure increases after removal of interstitial fluid, eventually causing the eruption rate reduction [9].
The researchers believe that a major role is played by differentiation of the tooth germ tissues, when the volume increases and certain pressure (tension) is generated in it under the force during eruption [10].
The developing mandibular third molar must be through the cascade of mineralized and non-mineralized connective tissues, and the enamel provides an insulating barrier that protects the tooth against physical, chemical, and thermal exposures under favorable conditions. In some cases the dental enamel structure insufficiency can make the course of dental disease more severe. There is an opinion that dental enamel contains no collagen, however, there are sporadic reports that provide evidence of the presence of collagen in this unique biological tissue [11, 12].
The complex epithelial-mesenchymal interactions and crosstalk within hard tissues take place at the early stages of tooth formation [13]. Recent observations make it possible to challenge conventional scientific evidence and assume that the enamel crystal growth is initiated by the mineralized collagen fibrils from dentin. Then these crystals cross the dentin–enamel junction to reach the ameloblast membrane and spread across the enamel surface. Such structure makes it possible to build the connective complexes, in which secretory ameloblasts form a semi-permeable barrier for intercellular transport of minerals and ions that circulate freely in the enamel matrix. Intercellular transport of fluids that neutralize pH of the enamel matrix can be realized through the above connective complexes [14].
The presence of collagen fibrils in the dental hard tissues is of significant scientific and practical value for dentistry. It has been found that in humans, amelogenins and enamelins interact with the collagen family members during intraosseous eruption [13]. Collagens are produced by odontoblasts and are found in the dentin, while amelogenins are produced by ameloblasts and are contained in the enamel. It has been shown that type IV collagen is expressed within the dentin– enamel junction, type I and VII collagens come from dentin and cross the dentin–enamel junction to reach the enamel. The role of the amelogenin–collagen and enamelin–collagen interactions or expansion of dentinal collagens into the enamel inner matrix will help explain the importance and unusual structural and biochemical features of the dentin–enamel junction [15].
Thanks to the development of dentistry, biochemistry, nanotechnology, new protein, dentin, and bone species have been reported that possess unique capabilities of affecting the hard tissue mineral structure. Microscopy results are indicative of the complex relationship between the apatite crystals; the issues of intermolecular and intramolecular interactions between protein matrices and the maxillofacial region mineralized tissue inorganic phase are the most pressing [16].
It is well known that inorganic phase of the dental enamel and dentin is represented by hydroxyapatite crystals showing more stable vibrations in the mid-IR region [12].
The literature reports studies of proteins contained in human fetal tooth germs and mature enamel of permanent teeth. Such studies cover just a few frequencies or IR spectra of proteins, while interpretation of the studies can be found in sporadic reports. More recent studies show that dental enamel of the fetal tooth germ is not similar to the erupted tooth enamel. The tooth germ enamel contains thousands of times less protein than the permanent tooth enamel, it differs little from soft tissues of the body in terms of density and protein content [17].
It has been found that the components of organix matrix originate from the dentin–enamel junction, where it partially intertwines with the dentin collagen fibrils along the whole length. The organic matrix that is above the dentin–enamel junction is represented by the layer of intertwined fine fibers forming something like an inhomogeneous wide-loop mesh along the whole length of the dentin–enamel junction separating enamel from dentin. Such a complex structure allows many researchers to affirm that the dentin collagen fibrils intertwine with the enamel organix matrix [18]. This, in turn, results to the emergence of collagen among specific enamel proteins, amelogenins and enamelins, while the organic matrix diffraction pattern is determined by the diffusely blurred rings.
The other studies demonstrate the decrease of the organic phosphate levels in the fetal dental enamel and mature enamel with age, which is reflected in the decrease in the IR spectra absorption bands. IR spectra of fetal dental enamel differ considerably from the collagen and keratin spectra, which makes it possible to consider the majority of enamel proteins to be collagen or keratin type proteins [19].
During enamel formation, intensity of the protein bands that are within the absorbance region of carboxyl groups is reduced in the IR spectra due to binding of mineral components. The content of orthophosphate groups in the fetal tooth germ enamel increases during formation of the apatite structure, while in mature enamel, the content of orthophosphate groups almost does not change with age. Intensity of vibrations of pyrophosphate groups contained in apatite of the enamel increases during pre- and postnatal ontogenesis [20].
Based on the reviewed literature, we believe that it is relevant and desirable to describe new ideas about the mandibular third molar eruption on the basis of biochemical and morphological rearrangements in these teeth.
The study was aimed to explore variability of the hard tissue organic matrix and mineral component in mandibular third molars being at different stages of tooth eruption using microscopy and biochemical methods.
METHODS
Clinical trial
The study involved 67 women aged 14–17, 18–21, 22–26, 27– 31, 32–36, who were followed up and treated by orthodontist and oral surgeon at the General Practice Dental Department of the City Clinical Dental Polyclinic №1, Center for Diagnosis and Treatment of Connective Tissue Dysplasia of the Omsk State Medical University (Omsk, Russian Federation). All study participants underwent extraction of teeth 38, 48 (due to orthodontic indications or tooth eruption difficulties) in 2021–2022. IR spectroscopy was performed in the Laboratory of Biochemistry Research of the Omsk State Pedagogical University.
Inclusion criteria for the study group: women, who underwent extraction of intact teeth (38, 48), with the confirmed diagnosis (according to ICD-10) of K05.22 (aggressive periodontitis), К05.32 (chronic periodontitis), К00.6 (disturbances in tooth eruption), К01.0 (embedded teeth); age 14–36 years; Slavic appearance (Caucasian race); connective tissue dysplasia; total score of +17 (severe degree). The comparison group included normosthenic women.
Exclusion criteria: male patients, women under the age of 14 and over the age of 36; mandibular third molars with chronic foci of infection in periapical tissues affected by caries; subcompensated or decompensated chronic disorder; individuals addicted to alcohol or drugs, those who had earlier used ulcerogenic drugs (non-steroidal anti-inflammatory drugs, glucocorticosteroids, etc.); hypersthenic body habitus; Asian or Negroid appearance; connective tissue dysplasia; total score of less than 17 (mild-to-moderate degree); the diagnosis not mentioned as an inclusion criterion (according to ICD-10).
The mandibular third molar eruption stages were assessed based on the computed tomography images and divided into four groups: tooth germ, tooth grown to the level of the gum, tooth grown to the mid-crown of the second molar, fully erupted tooth.
Connective tissue dysplasia was diagnosed, and dental hard tissues were collected for biochemical tests in accordance with the previously reported methods [21, 22].
Method to collect enamel, dentin–enamel junction, dentin for microscopy
During the first phase the extracted tooth was fixed with a woodworking clamp and separated in a mesiodistal direction with a diamond disc to provide a longitudinal section. The tooth fragment prepared was placed in the PVC tube chuck with the cut turned down and poured with the two part epoxy resin for 24 h.
During the second phase the resulting specimen was processed using the МР-1В Grinder/Polisher (MRC; UK) and the 800, 1500, 2000, 2500 grit dexter grinding wheels (Leroy Merlin; France). Final polishing was performed using the felt polishing wheel with the GOI polishing paste for plastics. The specimen surface was pickled with the 37% phosphoric acid (Н3РО4) for 20 s in the enamel area and for 15 s in the dentin area. The specimen was dried with the cellulose napkin. The specimen was placed on the stage of the Jeol JCM – 5700 scanning electron microscope (JEOL Ltd.; Japan) for examination. The use of the described method makes it possible to study the enamel, dentin, and dentin–enamel junction morphology on the longitudinal section using a scanning electron microscope.
Method of IR spectra acquisition and pre-processing
The enamel, dentin, and dentin–enamel junction samples were dried to the constant weight at a temperature of 105 °С for 6 h, then the mass fraction of moisture was determined. Powders were tested in tablets pressed in a mixture with potassium bromide (ratio 1 : 100; diameter 3.5 mm). The spectrum of pure potassium bromide previously dried at a temperature of ~600 °С for 6 h was used as a reference spectrum. IR absorption spectra were acquired with the FT-891 Fourier transform infrared spectrometer (SIMECS; Russia) in the 500–4000 cm–1 range (number of scans — 32, resolution — 4 cm–1). Baseline correction and normalization of spectra were performed using the ZaIR 3.5 software (SIMECS; Russia). Position and intensity of the absorption bands (AB) were determined in all spectra.
Statistical data processing
Statistical analysis of the results was performed using the Statistica 10.0 software package (StatSoft; USA) by nonparametric methods: Wilcoxon signed-rank test for dependent samples and Mann–Whitney U test for independent samples. The sample was described using median (Ме) and interquartile range in the form of the 25th and 75th percentiles (LQ; UQ). The differences were considered significant at p ˂ 0.05.
RESULTS
Our findings have shown that dentinal tubules originate on the dentin inner surface and cross dentin in the outward direction perpendicular to the tangential fibers of the ground substance. The radial and tangential collagen fibers define topographic arrangement and correct orientation of the dentinal tubules. It has been noted that in young people (aged 14–17, 18–21), the dentinal tubules are small, in contrast to the age groups of 22–26 years, 27–31 years, 32–36 years. In the age group of 32–36 years the decrease in the dentinal tubule size is observed that is due to the reduced trophic function and dental hard tissue mineralization, obliteration and emergence of numerous denticles, which adversely affect metabolic processes and can results in the dental hard tissue diseases. As mandibular third molar moves towards the occlusal plane and full eruption, the size of dentinal tubules significantly increases in all age groups (14–17 years, 18–21 years, 22–26 years, 27–31 years) (p < 0.05) (tab. 1; fig. 1А–E).
Our studies have demonstrated the increase in mineralization of the dentinal tubule inner layer at the stage of mandibular third molar eruption to the mid-crown of the second molar in the age groups of 21–26 years, 27–31 years, which is consistent with the literature data on the dentinal tubules’ varying width [23]. The described ring of the dentinal tubule mineralization is narrower near the pulp and wider near the dentin–enamel junction (tab. 1; fig. 1А–E). Thickening of the dentin layer is reported in all the compared age groups, except for the group aged 32–36, in which its thickness remains unchanged with the mandibular third molar development and its growth to the mid-crown of the second molar. Dentinogenesis proceeds evenly and actively throughout all stages of tooth eruption, which is reflected by statistical analysis of the groups being compared (p > 0.05) (tab. 1).
The width of prisms in various areas of enamel changes with age and as mandibular third molar eruption proceeds. We have revealed a significant increase in the size of enamel prisms in individuals aged 27–31; no such increase is observed in individuals over the age of 32 (p > 0.05). The rapid increase in the size of prisms in deep layers of enamel was detected during such tooth eruption stages, as tooth germ and tooth grown to the level of the gum, in all groups being compared. The width increase in the enamel surface layer throughout early stages of the mandibular third molar eruption is far behind relative to deep layers in all age groups (p > 0.05) (tab. 2; fig. 1E–O).
We have found that growth of prisms in the enamel surface layer significantly increases in the age groups of 18–21 years (p = 0.001), 22–26 years (p = 0.002) during the stages of tooth germ and tooth grown to the level of the gum, while in deep layers it is increased in the age group of 22–26 years throughout all stages of tooth eruption (p < 0.05).
We have revealed significant differences in the mandibular third molar hard tissue water content that characterize metabolic processes and the rate of the molars’ maturation on their way to full eruption. The highest enamel water content is observed in the age groups of 18–21 years, 22–26 years at the stage of the mandibular third molar grown to the level of the gum. No significant changes in the enamel water content were revealed at other stages of tooth eruption (p > 0.05) (tab. 3).
The dentin water content changes with age. The highest dentin water content is observed in the age groups of 18–21 years, 22–26 years throughout all phases of tooth eruption (p < 0.05). A significant increase in water content associated with the mandibular third molar full eruption is observed in the above age groups. No significant differences have been revealed in other age groups, however, the water content downward trend has been reported for the age group of 32–36 years (tab. 3).
The same changes in this parameter as that reported for dentin have been reported for the dentin–enamel junction, along with positive dynamics of the increase in the age group of 22–26 years throughout all phases of tooth eruption (tab. 3).
A total of 19 absorption bands have been identified in the mandibular third molar hard tissue IR spectra: 3239 cm–1 (νsO-H); 2963 cm–1 (νasCH3); 2922 cm–1 (νasCH2); 2855 cm–1 (νsCH2); 1769 cm–1 (νC=C); 1637 and 1618 cm–1 (νC=O); 1546 cm–1 (δN-H, νC-N); 1454 cm–1 (δasCH3, δCH2); 1418 cm–1 (νC-N, δN-H, δC-H); 1384 cm–1 (δCH3); 1342 cm–1 (CH2); 1249 and 1202 cm–1 (δN-H, νC-N); 1106 cm–1 (νsPO, νCC, νCO); 1050 cm–1 (PO4); 1037 cm–1 (νCC, νCO, νCH2OH); 967 cm–1 (νPO4); 876 cm–1 (δO-C-O) [22].
It has been shown that the set of absorption bands found in the mandibular third molar hard tissue IR spectra qualitatively does not change with age, while intensity of certain absorption bands changes significantly (fig. 2). Intensity of the absorption bands of the methyl and methylene groups of lipids and proteins decreases with age (2855, 2922 and 2963 cm–1), while that of phosphate groups increases (1050 cm–1). This suggests the higher degree of hard tissue mineralization in the age groups of 27–31 years, 32–36 years. Apparent changes in the dentin–enamel junction structure predominate among age groups (fig. 2B). Intensity of the dentinal collagen absorption bands (1202, 1249 and 1342 cm–1) decreases with age (fig. 2C), while that of the enamel increases (fig. 2А); the dentin–enamel junction occupies an intermediate position and combines the decrease in the 1202 cm–1 band intensity with the increase in the 1342 cm–1 intensity (fig. 2B). Such findings demonstrate an important role of the dentin–enamel junction being a key link between the enamel and dentin due to metabolic, shock-absorbing, protective, and nutritional functions.
The principal component analysis of the enamel, dentin and dentin–enamel junction samples’ IR spectroscopy results in different age groups has shown that no differentiation of groups is reported for enamel and dentin. The vertical axis partially separates the age groups of 14–17 years and 27–31 years from other groups, however, such differentiation is non-significant (р = 0.5298 for enamel and р = 0.4157 for dentin) (fig. 3А, B). The opposite pattern can be observed for the dentin–enamel junction (fig. 3C, D). It is clear that the age groups of 14–17 years and 27–31 years are to the left of the vertical axis, while the horizontal axis separates these groups from each other (fig. 3C). In this case differentiation of the groups is significant (p = 0.0392). The absorption bands of phosphate ions (1050 cm–1; r = 0.7843; p < 0.0001), methyl and methylene groups of the lipid or protein carbon skeleton (2963 cm–1; r = 0.7268; p < 0.0001), (2855 cm–1; r = 0.6967; p < 0.0001), and collagen (1202 см–1; r = 0.6592; p < 0.0001) (1249 cm–1; r = 0.4763; p < 0.0001) contribute the most to differentiation of age groups (fig. 3D), as we have earlier reported. A negative correlation coefficient has been obtained for the 1037 cm–1 (r = –0.8105; p < 0.0001) and 1418 cm–1 (r = –0.6498; p < 0.0001) absorption bands. As for the second axis, maximum contribution has been reported for the 1454 cm–1 absorption band (r = 0.7371; p < 0.0001) corresponding to vibrations of the methyl and methylene groups of proteins and the 1249 cm–1 collagen absorption band (r = –0.4117; p < 0.0001) (fig. 3D).
The differences in the enamel, dentin, and dentin–enamel junction have been demonstrated at various stages of the mandibular third molar eruption. No significant changes in enamel have been revealed at the tooth germ stage, biochemical processes represent an integrated process. The mandibular third molar movement is associated with significant changes. The 0–0 horizontal axis separates the group with the teeth erupted to the middle of the second molar (above the axis) from the groups with fully erupted teeth (below the axis). The 0–0 vertical axis divides the groups with fully erupted teeth and the groups that are in the tooth germ phase. A more clear delineation of the groups is observed for dentin and dentin–enamel junction.
DISCUSSION
When comparing IR spectra in the comparison groups we agreed that orientation and organization of the collagen fibrils in dentin at early tooth eruption stages defined correct enamel prism packaging. Strong dentin-to-enamel adhesion is achieved due to correct organization of the dentin–enamel junction via a complex system of the tangling collagen fibrils in it [24]. The dentin biochemical alterations can results in abnormal enamel prism maturation, especially at early stages.
It has been noted that collagens are expressed in the dentin–enamel junction and dentin in all age groups. The significance of such interactions, as amelogenin–collagen and enamelin–collagen, as well as the expansion of dentin collagens into the inner matrix of the deep enamel layer help explain structural, biochemical, and morphofunctional changes of enamel after the mandibular third molar eruption [25, 26].
We have found that sufficiency of the dentin–enamel junction and dentin connective tissue complex provides a strong semipermeable barrier for intercellular transport of minerals and ions that circulate freely in the enamel matrix, thereby enabling a faster and more full-fledged maturation of mandibular third molars. Our observations complement the data of foreign researchers on the enamel crystal growth induced by the collagen fibrils of the dentin–enamel junction and dentin [27]. The enamel heterogeneity and integrity depend on the dentin–enamel junction and dentin biochemical and morphological state. The presence of congenital or acquired disorder or condition can impair the collagen structure, thereby causing major alterations of the dentin–enamel layer and enamel [28, 29].
The facts, that clinical material was collected from residents of one region only (Omsk Region), from women, mandibular third molars were the object of the study and no other groups of teeth were studied, and the samples of patients in the studied group were small, can be considered the study limitations. This necessitates further research in this area.
CONCLUSIONS
The results of the mandibular third molar hard tissue morphological and biochemical assessment confirm the existing data that mandibular third molars start changing their position at the tooth germ stage, when the pronounced changes in packaging and orientation of the dentin–enamel junction and dentin collagen fibrils and fibers, as well as their expansion into deep enamel layers affect initial spatial orientation and topographic arrangement of these teeth in the mandible. The data reported can answer the key question that correct orientation of the collagen fibrils in dentin and the dentin–enamel junction, coordinated action of the drainage network between the enamel and dentin via intraprismatic and interprismatic spaces and dentinal tubules that co-produce and increase pressure in the coronal part of the tooth trigger movement and growth of the mandibular third molar germs. This is a working hypothesis that needs in-depth analysis and requires further research.