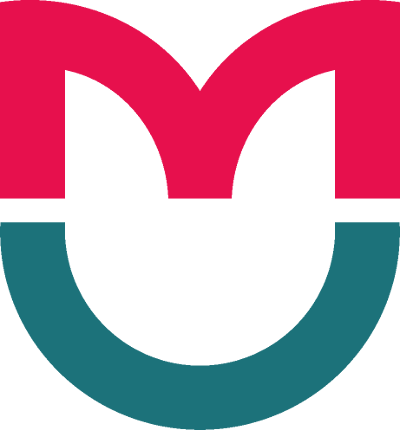
ORIGINAL RESEARCH
Effect of probenecid on astrocyte activation in vitro
1 Pirogov Russian National Research Medical University, Moscow, Russia
2 Lomonosov Moscow State University, Moscow, Russia
Correspondence should be addressed: Lyubov R. Gorbacheva
Ostrovitianova, 1, Moscow, 117997, Russia; ur.liam@76ibrog
Funding: The study was supported by the Russian Science Foundation, Project № 22-25-00848.
Author contribution: Babkina II, Morozova MP — obtaining and maintaining primary culture of astrocytes; collection, interpretation and statistical analysis of data, manuscript writing; Mazeeva VV — obtaining and maintaining primary culture of astrocytes, interpretation and statistical analysis of data; Gorbacheva LR — conception and design of the experiment, interpretation of data, project management, manuscript writing.
Compliance with ethical standards: the study was approved by the Ethical Committee of Pirogov Russian National Research Medical University (Protocol № 23/2021 dated December 13, 2021).
Inflammatory response is aimed at removing the damaging agent and restoring the structural and functional integrity of the tissue and can be accompanied by both pathogenetic and adaptive changes. Triggering of an acute inflammatory response in the nervous tissue can activate the processes of neurogenesis, angiogenesis and functional plasticity of neurons. However, the chronic inflammation potentiates further cell alteration and exacerbates neurodegenerative diseases increasing the risk of complications and disabilities.
Astrocytes are among the most abundant CNS cells involved in neuroinflammation. The level of astrocyte activity largely determines the outcome of neuroinflammation. Activated resident and immunocompetent CNS cells including microglia and astrocytes are the source of proinflammatory factors. As a result, there is an increase in BBB permeability, infiltration of immune cells into brain tissue, additional alteration, activation of hemostasis system and thrombin formation.
Development of neurodegenerative processes was shown to be associated with thrombin-dependent enhancement of microglia activation and is mediated by iNOS stimulation, secretion of reactive oxygen species (ROS) and proinflammatory factors COX-2, TNFα, IL1β, IL6 [1]. In addition, thrombin potentiates astrocyte proliferation through activation of PAR1/ MARK cascade.
Neuroinflammation can also be triggered by exogenous factors such as lipopolysaccharides (LPS), a component of bacterial cell wall. The main target of LPS is TLR4 receptor expressed by astrocytes, microglia and endothelium, and its activation triggers the formation of proinflammatory mediators.
The channels formed by pannexin proteins play an important role in the development of the inflammatory response involving astrocytes. The pannexins not only provide transport but also form complexes with other ionic and metabotropic receptors regulating the activity of these receptors [2]. A special role is played by pannexin 1 (Panx1) which is widely present in the CNS and expressed by neurons, astrocytes, microglia, cerebral endothelium and smooth muscle cells [3]. Panx1 contributes to development of neurodegeneration by regulating the release of ATP and other nucleotides from cells, inflammasome assembly and cytokine secretion. Panx1 is thereby a potential target for pharmacotherapy of neurodegenerative diseases and acute brain injuries [4, 5].
Probenecid having a broad spectrum of action including Panx1 inhibition, might be a potential neuroprotective agent. It has been shown that probenecid can specifically activate TRPV2 in sensory neurons and block ATP release from both astrocytes and microglia [5, 6]. Therefore, it can be considered as a promising drug for treatment of neurodegenerative and psychiatric diseases.
The aim of the present study was to evaluate the effect of probenecid, a Panx1 blocker, on thrombin- and LPS-induced proinflammatory activation of mouse astrocytes in vitro.
METHODS
Reagents
10× HBSS without Ca2+ and Mg2+ (Gibco; USA), 1M HEPES (Gibco; USA), 10× PBS (Gibco; USA), BSA (Sigma; USA), DMEM/F12 cell culture medium (Gibco; USA), inactivated fetal bovine serum HI FBS (Gibco; USA), penicillin-streptomycin (Invitrogen; USA), trypsin-EDTA (Gibco; USA), Versen solution (PanEco; Russia); probenecid (BioQuest; USA); thrombin (Sigma; USA), lipopolysaccharide Escherichia coli O111: B4 (L3024; Sigma, USA), Griess reagent (Sigma; USA), Cell Proliferation Reagent WST-1 (Sigma; USA), Mouse IL6 ELISA kit (abcam ab 222503), Triton×100 (Sigma; USA), RIPA lysis buffer (Sigma; USA), Complete Protease Inhibitor Cocktail (Roche; USA), Bradford reagent (Bio-Rad; USA).
Obtaining and maintaining primary astrocyte culture
Primary cortical astrocytes were isolated from the cerebral hemispheres obtained from 0 to 3-day-old C57BL/6 mice. Cell cultures were prepared as described in [7]. Brain hemispheres were extracted and placed in buffer (1× HBSS, 100 mM sodium pyruvate and 1M HEPES), washed and cut into pieces. The brain homogenate was incubated with 0.5 mg/mL papain (1× PBS, L-Cystein-HCl, BSA, glucose, 8 min, 37 °C). The homogenate was then resuspended in buffer containing DNase (0.01 mg/mL) followed by centrifugation at 1,500 g for 5 min at +4 °C. The resulting precipitate was resuspended in buffer (1× HBSS with Ca2+ и Mg2+, 100 mM sodium pyruvate, 1M HEPES) and recentrifuged. The precipitate was resuspended in 1 ml of culture medium (DMEM/F12, 10% FBS, antibioticantimycotic, GlutaMAХ). Cells were placed in 25 cm2 culture flasks and cultured for 10–12 days at 37 °C and 5% СО2. Complete medium replacement was performed on day 2 and day 7 by removing microglia, placing flasks with cells in a shaker for 6–8 h, with further medium replacement with a fresh one. Four days before starting the experiment, the cells were removed from the flasks by 0.05% trypsin solution in phosphate buffered saline and placed in 48-well plates. Before experimental exposure, the culture medium was replaced with serum-free medium for 3 h followed by addition of thrombin at a final concentration of 50 nM or 100 nM or LPS at a concentration of 100 ng/mL or 1 μg/mL. Probenecid 0.1 mM was added 30 min before thrombin or LPS exposure. Measurements were performed 6 h, 24 h, and 48 h following exposure.
Astrocyte survival assessment (WST-1 Assay)
Cell survival was assessed 24 h after astrocyte activation using the WST-1 test according to the manufacturer's protocol. Optical density was measured at λ = 450 nm using iMark™ microplate absorbance reader (BioRad; USA). The obtained data were interpreted as cell proliferation, assuming that contribution of cellular hyperplasia under these conditions is not significant, and guided by the manufacturer’s information presenting this test as WST-1-proliferative reagent.
Assessment of nitrite accumulation in astrocyte culture medium
Nitric oxide (NO) secretion was assessed by nitrite accumulation in the culture medium 24 h after incubation with inflammatory inducers, using the Griess reagent. The Griess reagent forms colored nitrogen compounds when interacting with nitrite — the absorbance intensity of the compounds was evaluated at 530 nm using iMark™ microplate absorbance reader (BioRad; USA). The results were presented in relative values as NO secretion by one cell using the ratio of nitrite level in the culture medium to the number of cells in the corresponding well estimated by WST-1 assay.
Assessment of β-hexosaminidase activity
β-Hexosaminidase (HEX) activity was assessed 24 and 48 h after astrocyte activation using the modified method proposed by Schwartz LB et al. [8] in supernatant and intracellularly for subsequent calculation of enzyme secretion as follows:
A (A + B) × 100%,
where A is the optical density of the sample reflecting the enzyme activity in the culture medium minus the background optical density; B is the optical density of the sample reflecting the activity of the intracellular pool of enzyme minus the background optical density.
Further, HEX secretion was represented in relative units in relation to control taken as 1.
Assessment of IL6 secretion by astrocytes
IL6 secretion by astrocytes 6 h after exposure was assessed using Mouse IL6 ELISA kit (abcam, Cat. № ab222503) according to the manufacturer's protocol. The sensitivity limit of IL6 was 30 pg/ml. IL6 content was determined in exposed culture medium samples using the kit. Cells in the wells were washed with phosphate buffered saline and lysed with RIPA buffer with protease inhibitors. Lysates were centrifuged at 14,000g for 15 min at 4 °C, after that total protein content was measured in the supernatant using the Bradford kit. IL6 secretion was represented in pg/mg of total protein.
Statistical Analysis
Statistical analysis was performed using GraphPad Prism 8 (GraphPad Software Inc.; USA). Normality of sample distribution was assessed using the Shapiro–Wilk test. To compare groups, two-factor ANOVA analysis of variance followed by a posteriori analysis using Tukey's criterion was applied. Data were presented as arithmetic mean ± standard error (SEM), differences were considered statistically significant (*, #) at p < 0.05. The number of experiments (n, number of astrocytes plantings) was indicated in each case separately.
RESULTS
Effect of probenecid on the survival of thrombin- and LPS-activated astrocytes
Thrombin is involved in the inflammatory response since the hemostasis system in the first place reacts to tissue damage. In the first series of experiments, neuroinflammation was modeled by adding thrombin to the culture medium of mouse astrocytes. It was demonstrated that thrombin at a concentration of 100 nM significantly (p < 0.05) increased the number of living astrocytes, while treatment of cells with probenecid reduced this index to control values which may indicate the participation of Panx1 channels in thrombin-induced astrocyte proliferation (fig. 1). In contrast to thrombin, LPS caused a decrease in survival under the same conditions at all concentrations used, which may indicate its toxic effect on astrocytes (fig. 1). Probenecid did not alter the effect of LPS (fig. 1).
Effect of probenecid on NO secretion by activated astrocytes
Increased NO production is a sign of neuroinflammation. In this study, it was observed that 24 hours after application of 100 nM thrombin (not 50 nM), there was a significant accumulation of nitrites in the culture medium of astrocytes while probenecid stopped thrombin-induced NO secretion, indicating the Panx1 involvement in this process (fig. 2). In case of 24-hour incubation of cells with LPS, the most significant increase in NO secretion was observed at 0.1 μg/mL LPS concentration which was terminated using Panx1 blockade. In case of 1 μg/mL LPS concentration, NO secretion did not differ from control values and did not depend on Panx1 (fig. 2).
Effect of probenecid on β-hexosaminidase secretion by activated astrocytes
Release of the lysosomal enzyme β-hexosaminidase (HEX) from cells may also be considered as a marker of proinflammatory activation of astrocytes.
The effect of probenecid on HEX secretion by astrocytes was assessed 24 and 48 h after their activation by thrombin (concentrations 50 nM and 100 nM) and LPS (concentrations 0.1 μg/mL and 1 μg/mL). After 24 h we observed the proinflammatory effect of thrombin at the concentration of 100 nM and the involvement of Panx1 in the realization of this effect since its blockade by probenecid significantly reduced HEX secretion (fig. 3). Application of 0.1 μg/mL LPS caused cell activation after 24 h, in contrast to the effect of 1 μg/mL LPS (fig. 3). In both cases, blockade of Panx1 channels by probenecid did not change the effect of endotoxin (fig. 3).
The increase of thrombin exposure time from 24 to 48 h resulted in a more significant increase in HEX secretion by astrocytes and was mediated by Panx1 since this index decreases to control values when probenecid was applied (fig. 4). HEX secretion on the background of LPS, similarly to thrombin, was higher 48 h after exposure. At the same time, the anti-inflammatory effect of probenecid was manifested only against LPS at a concentration of 1 μg/mL — HEX secretion decreases to control level (fig. 4).
Effect of probenecid on IL6 secretion by activated astrocytes
Astrocyte activation is associated with the secretion of proinflammatory cytokines, particularly IL6. High concentrations of thrombin and LPS increased the levels of IL6 secretion 6 h after induction. Effect of LPS was 2-fold more significant compared with the effect of thrombin (p < 0.05). Thus, LPS appeared to be a more specific proinflammatory agent. Probenecid in both cases had an anti-inflammatory effect and stopped the proinflammatory activation of astrocytes caused by both thrombin and LPS, significantly reducing the level of IL6 secretion (fig. 5) and indicating the involvement of Panx1 in secretory function of reactive astrocytes.
DISCUSSION
Chronic neuroinflammation is a pathogenetic factor that triggers neurodegeneration processes and increases the risk of irreversible CNS damage. Astrocytes, the most abundant brain cells contributing to neuron-glia interaction, may be involved in regulating neuroinflammation. Proinflammatory activation of astrocytes is associated with an increase in their proliferative and secretory activity and may be accompanied by opening of Panx1 channels [9].
Releasing ATP from cells including ATP release through Panx1 channels is a signal for a variety of processes starting from migration, proliferation and growth in physiological conditions to triggering neuroinflammation and neurodegeneration in pathological conditions. ATP effects are realized through purinergic receptors — ligand-gated ion channels (P2X) and G protein-coupled metabotropic receptors (P2Y). P2X7 receptors play an important role in triggering neuroinflammation. Panx1 blockade by probenecid can limit ATP release from cells and — mediately — purinergic signaling [6]. However, probenecid was also reported to be able to block P2X7 receptors directly [10].
Interestingly, Panx1 co-expressed with P2Y1 and P2Y2 receptors via PLC-cascade caused an increase in intracellular Са2+ levels which enhanced Panx1 activation [11]. Calciuminduced activation of Panx1 supposedly occurred through CaMKII phosphorylation, Panx1 opening and ATP release [12].
In brain ischemia and excitotoxicity, NMDAR overactivation occurs which activates Panx1 and triggers proapoptotic signaling pathways [13]. Panx1 activation initiates the assembly of inflammasomes, multimeric protein complexes in the cytosol of all cells that control the inflammatory response. Inflammasome activation provides an active form of proinflammatory caspase-1 cleaving pro-IL1β and pro-IL18 to form mature cytokines IL1β and IL18 [3, 6]. Pyroptosis, a pro-inflammatory form of cell death accompanied by release of pro-inflammatory signals, is also caspase-1-dependent. ATP release, e.g. via Panx1, into the environment and subsequent activation of P2X7R also results in activation of inflammatory processes, inflammasome assembly and IL1β production [6].
In a neuron-astrocyte co-culture system, ATP and glutamate released from astrocytes treated with conditioned medium from activated microglia, were shown to cause neuronal death through activation of Panx1 neurons [14]. Panx1 regulated the release of ATP and other nucleotides, inflammasome assembly and cytokine secretion, which may determine its contribution to neurodegeneration. Panx1 is therefore a potential target for pharmacotherapy of neurodegenerative diseases and acute brain injuries. Possible conjugation of PAR1 with Panx1 channels was described in [15].
In this study, we demonstrated that quantity of astrocytes increased under the action of thrombin (fig. 1). Such effect may be associated with thrombin/PAR1-mediated phosphorylation of ERK1/2 or activation of PLCε, which in turn regulates MAPK/NFκBintracellular cascade [16]. This effect may also be associated with triggering thrombin/PAR1-dependent IL6 secretion by astrocytes and further signaling through the IL6/STAT3 pathway, development of astrogliosis including increased levels of glial fibrillary acidic protein (GFAP) and vimentin. In the present study, probenecid was shown to inhibit the thrombin-mediated increase in the number of astrocytes and to return this index to control values indicating that Panx1-channels may be involved in this process. This is consistent with the data obtained for lung epithelial cells and human endotheliocytes where thrombin-dependent activation of Panx1 channels was demonstrated [15, 17]. However, the mechanisms of such cooperation are still unknown.
LPS proinflammatory factor is widely used to model the inflammatory response. LPS-induced proinflammatory activation is known to be mediated by TLR4. TLR4 triggers activation of a complex of factors including NF-κB through MyD88-IRAK-TRAF6-TAK1 signaling complex [18]. As a result, astrocyte proliferation may be altered and pro-inflammatory cytokines may be expressed through MAPK/NF-kB activation [19]. In addition, it was shown that the Akt/ERK/JNK cascade may be involved in LPS-mediated inflammation since its blockade decreased the secretion of proinflammatory cytokines [20]. Some data is available on LPS/TLR4-dependent increase in the level of mouse astrocyte proliferation.
In the present study, LPS was shown to reduce cell survival but activate the secretion of primary cultured mouse cortical astrocytes. LPS-induced activation of astrocytes expectedly resulted in a persistent increase in secretion of the proinflammatory interleukin IL6 (fig. 5) and, to a less extent, NO and HEX (fig. 3, fig. 4). Remarkably, blockade of Panx1 channels by probenecid significantly reduced LPS-induced proinflammatory secretion of astrocytes.
Proinflammatory activation of astrocytes is accompanied by an increase in production of many chemokines and cytokines as well as activation of inducible NO synthase (iNOS) and NO secretion [21, 22]. Increased NO levels are shown to accompany many neurodegenerative diseases such as Alzheimer's and Parkinson's diseases and can also contribute to formation of reactive nitrogen species having cytotoxic effect on neurons [23, 24]. In astrocytes, both endogenous and exogenous proinflammatory factors can act as inducers of NO production [21, 24]. We have observed an increase in NO secretion by primary mouse astrocytes under the action of thrombin and LPS (fig. 3). PAR1-mediated NO secretion and iNOS activation were previously demonstrated on astrocytoma cells [25]. The observed thrombin-induced NO production by cultured astrocytes reduced under Panx1 blockade with probenecid (fig. 3). This may be an evidence of possible interaction between thrombin receptor PAR1 and Panx1. Activation of Panx1 channels can be induced by high concentration of intracellular Са2+ which, in turn, can be a consequence of the thrombin/PAR1/Gq/RCS/IP3 signaling pathway activation [4]. Panx1-dependent ATP release via P2X7 enhances thrombininduced increase of intracellular calcium [26] and activation of nNOS and eNOS in astrocytes.
We have demonstrated that LPS-stimulated NO secretion by astrocytes as well as thrombin-induced NO secretion stopped in the presence of probenecid (fig. 2). Panx1-dependent effects of LPS on HK-2 cells were described in other investigations. In particular, under Panx1 inhibition, LPS-induced inflammation was alleviated with inhibition of NLRP3 and decreased expression of Bax and Bcl2 [27].
Level of activation of immunocompetent cells (macrophages, mast cells) can be estimated based on HEX secretion level. In our study, we used substrate-enzyme reaction to evaluate the activity of HEX in astrocyte cultivation medium in 24 h and 48 h after thrombin and LPS application. Both in case of thrombin and LPS, the increase in HEX secretion was more significant after 48 hours that can probably be explained by delayed activation of astrocytes on the principle of positive feedback. These results are consistent with the data on thrombindependent increase in HEX secretion in thrombocytes and mast cells demonstrating the involvement of P2X7 receptors in activating degranulation [28].
Proinflammatory cytokine IL6 is one of the specific markers of inflammation. Analysis of IL6 level in the astrocyte cultures confirmed the proinflammatory effect of both thrombin and, to a greater extent, LPS (fig. 5). Similar proinflammatory effect of thrombin was previously observed for astrocytes [29]. This protease effect is attributed to thrombin/PAR1-dependent activation of G12/13 protein triggering the RhoGEF/RhoA/
phospholipase Cε — DAG and PKС — PKD cascade, culminating in increased expression of IL6, COX-2 and other proinflammatory genes [30]. Clear proinflammatory effect of LPS on astrocytes with induction of IL6 secretion was also demonstrated by other researchers [31]. In the present study, we have shown for the first time a probenecid-dependent decrease in thrombin- and LPS-induced IL6 production for primary astrocyte cultures (fig. 6). Probenecid-induced inhibition of TNFα, IL6, IL1β and IL8 expression including in the presence of LPS was observed by Z. Zhang et al. on hippocampal cells in sepsis, and by L. Wei et al. on U87-MG cells [19, 32]. At the same time, the results of some other investigations do not confirm the participation of Panx1 and potential heterodimers P2X4/P2X7 in P2X7-dependent release of IL6, CCL2 and TNFα in microglia [33].
We investigated the effects of probenecid having a broad spectrum of activity as a potential neuroprotective agent. Owing to its high lipid solubility, probenecid freely penetrates to the BBB. It is able to interact with TRPV2 membrane protein channels and organic anion transporters (OAT1-3) and cation transporters (OST1-3) as well as Panx1 channels which indicates its potential therapeutic use, e.g. as an adjuvant to increase the bioavailability of some drugs in the CNS. Using probenecid as a blocker of Panx1 channels seems to be promising to suppress neuroinflammation, a neurodegenerative component of many CNS diseases [6]. With a great number of Panx1 activating stimuli and functionally diverse effects, probenecid exhibits dual activities — on the one hand, it may trigger anti-inflammatory effects by inhibiting inflammasome activation while on the other hand, reduced glial-neuronal interaction and plasticity increases the risk of additional tissue damage. Decreased levels of AQP4, NLRP3 and caspase-1 expression in astrocyte culture under oxygen-glucose deprivation evidences about anti-inflammatory and neuroprotective effect of probenecid [34]. Probenecid increased astrocyte survival by reducing ROS production and suppressing the expression of NLRP3, caspase-1, and IL1β [6].
Thus, using Panx1 inhibitor probenecid as an antiinflammatory agent (fig. 6) may be considered for developing new approaches for effective control of neuroinflammation, one of the important factors potentiating brain damage in trauma and neurodegenerative diseases [35, 36].
CONCLUSIONS
Proinflammatory activation of mouse astrocytes by application of thrombin (50 nM, 100 nM) and lipopolysaccharide (0.1 μg/mL, 1 μg/mL) changed their functional profile as well as cell proliferation and secretory activity. LPS induced a significant increase in secretion of IL6 while thrombin had a more significant effect on NO production and astrocyte proliferation. The induced production of NO, HEX and IL6 was observed to be terminated by the Panx1 channel blocker probenecid. Remarkably, probenecid abolished the effect of thrombin on astrocyte proliferation but not the effect of LPS. Inhibition of IL6 and HEX secretion by probenecid also differed in case of LPS as compared with thrombin. The obtained results indicate the possible involvement of Panx1 channels in thrombin- and lipopolysaccharideinduced proinflammatory activation of astrocytes and induction of astrogliosis. Identifying mechanisms and key participants of the signalling cascade triggered in thrombin- and LPS-induced neuroinflammation under pretreatment of cell cultures with probenecid is subject to further investigation.