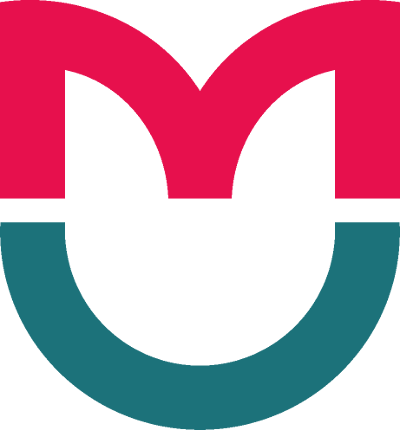
ORIGINAL RESEARCH
A novel spheroid model for preclinical intercellular nanophotosensitizer-mediated tumor study
1 Prokhorov General Physics Institute of the Russian Academy of Sciences, Moscow
2 Research Centre for Automatic Control of Nancy, University of Lotaringia, Nancy, France
3 The Belarusian State University, Minsk, Belarus
4 National Research Nuclear University MEPhI, Moscow
Correspondence should be addressed: Yuliya S. Maklygina
Vavilova 38, Moscow, 119991; ur.usm.scisyhp@avonosmas.su
Funding: this work was supported by the Ministry of Education and Science of the Russian Federation (Agreement RFMEFI61618X0096 # 14.616.21.0096 of February 12, 2018).
Nanoparticles (NPs), which are based on molecular nanocrystals of photosensitizer (PS), are promising agents for the fluorescence diagnostics (FD) and treatment by the photodynamic therapy (PDT). Aluminium phthalocyanine (AlPc) nanocrystals have an advantage over the molecular PS used in clinic settings due to the significantly higher accumulation selectivity of nanoscale materials [1–4]. Moreover, they are able to fluoresce only in monomeric form upon the interaction of nanocrystals with biological structures, hereby providing appropriate FD detection efficiency [1, 2]. The type of interaction, the intensity, the lifetime and the spectrum of fluorescence depends on phenotype of the interacting cells. Fluorescence intensity of AlPc NPs in pathological tissue (inflammation, malignancy) significantly exceeds that in normal tissue [1, 2]. Moreover, AlPc NPs can be considered as theranostic probes providing both fluorescence for FD and photosensitizing activity for PDT treatment.
The in vitro screening of novel anti-cancer agents, particularly PSs, is mainly relied on photocytotoxicity assays using established cancer cell lines. Conventional two-dimensional (2D) 2D cell cultures exhibit a rapid, uncontrolled growth phenotype and are not able to mimic the complexity and heterogeneity of in vivo tumors. Evidently, in vivo tumors grow in a three-dimensional conformation with a specific organization and architecture that a 2D monolayer cell culture cannot reproduce [5–7]. Three-dimensional (3D) cell cultures are considered as a more accurate and reproducible model for performing in vitro drug screening. This model displays several features of in vivo tumor tissues such as presence of extracellular matrix, intercellular interaction, hypoxia, drug penetration and resistance [8–10]. Therefore, in vitro spheroid model is an intermediate stage between conventional 2D in vitro testing and animal models. The sphe [11–13].
Thereby, we have chosen 3D multicellular spheroids as a model to study accumulation, distribution and PDT efficiency of AlPc NPs in HeLa cells.
METHODS
Multicellular spheroids were initiated by seeding 104 HeLa cells into 96-well plate previously coated with 1% Agarose. Spheroid culture medium was changed every 2–3 days. When spheroids reached 140 ± 20 μm in diameter after 7 days, they were used for experiments. In this study, aluminium phthalocyanine nanoparticles (AlPc NPs, d ~ 100 nm, c = 10 μg/ml) were used as the PS. The investigations of AlPc fluorescence after different incubation intervals were performed using laser scanning confocal microscopy. For microscopy the spheroids were finally washed twice with pre-warmed phosphate buffered saline (PBS). The images were acquired with laser scanning microscope LSM-710-NLO (Zeiss; Germany). The 20× Plan-Apochromat objective with numerical aperture (NA) of 1.4 was used. The novel PS Aluminum phthalocyanine (AlPc) (synthesized by Organic Intermediates & Dyes Institute (NIOPIK), Russia) was prepeared and studied using the spheroid model. The polycrystalline powder was added to distilled water to a concentration of 1 mg/ml. The resulting suspension was dispersed in Bandelin SONOPLUS HD2070 ultrasonic homogenizer with KE76 attachment (20 kHz, the amplitude of 165 microns) [2]. Using Photocor Complex (Russia) multi-angle spectrometer of the dynamic light scattering, allowing obtaining the nanoparticles distribution by size via the analysis of correlation function of the scattered light intensity fluctuations, it was found that the average particle diameter in the aqueous colloid was 100–150 nm. AlPc colloid (c = 10 µg/ml) was added to the medium of the spheroid model to mimic conditions of tumor cells interaction with PS NPs. The main AlPc NPs feature is the photoactivation ability. The primary AlPc colloid did not luminesce upon the laser excitation into the absorption band (at the wavelengths 633 nm and 780 nm by two-photon ex.) i.e. the PS nanocrystals in a free form showed no photoactivity. So, the nanoparticles colloid of AlPc was not initially photoactive and did not display fluorescent properties. However, during interaction of AlPc NPs with cells, the NPs are involving into metabolic cells processes and become photoactive (λfl ~ 670 nm at the excitation λex ~ 633 nm and 780 nm by two-photon ex.).
Thus, the experiment protocol consisted of the following steps (fig. 1.):
1) At the beginning of experiments, 10 spheroids have been transferred to separate Petri dish. After that the AlPc NPs colloid was added to a set of 10 spheroids at the concentration 10 µg/ml each. PS incubation was performed at 37 °C for 15 min in the dark.
2) During futher incubation the cells autofluorescence was excited with 488 nm laser and simultaneously the AlPc NPs fluorescence was excited with 633 nm laser under the laser scanning microscope. After 1 hour of AlPs NPs accumulation the fluorescence signal stopped rising.
3) After that, the spheroids were washed twice with PBS and directly observed on an upright fluorescence microscope. Fluorescence images were recorded using 20× objective from the spheroid surface. After PS NPs interaction with biocomponents the NPs photoactivity was sufficient for the FD and PDT. Therefore, the detected interest zones were exposed to laser radiation with wavelength 780/390 nm (by two-photon excitation) after analysis of PS accumulation.
4) Laser irradiations were performed at 780/390 nm (by two-photon excitation). The irradiation times were adapted for each irradiation. The assessement of photodynamic effect was realized analyzing the fluorescent signal after staining with acridine orange (for healthy cells detection — green; AO, MolecularProbes®) and ethidium bromide (for dead cells detection — red; EB, MolecularProbes®). For staining, the spheroids in PBS, previously washed from the culture medium, were incubated in the presence of working solution of dyes for 5 minutes. Stained spheroids from the 96-well plates for in vitro culture have been transferred to Petri dishes with a 0.17 mm glasse’s thick in PBS solution. AO/EB fluorescence signals distribution was studied using confocal microscopy. Excitation of fluorescent AO was performed with a 488 nm laser, fluorescence was recorded in the range of 495–545 nm. Excitation of fluorescent EB was performed with a 561 nm laser, fluorescence was recorded in the range of 580–690 nm. As a result, fluorescence images of AO (green) and EB (red) were obtained in the transmitted light mode. Thus, this stepwise approach enabled mimicing the conditions of tumor cells interaction with PS NPs at the first hour and the processes of FD and PDT with AlPc NPs in vivo.
RESULTS
AlPc NPs uptake in spheroids was evaluated at different times during 1 hour. An intense accumulation was observed during the first 30 minutes
(fig. 2 A–C). After 40 minutes of incubation the fluorescence signal reached a plateau without considerable further changes (fig. 2 D–F). AlPc NPs fluorescence flaring up visualization in space and time allowed tracking of PS distribution. It was observed that after first 15 minutes AlPc NPs were accumulated in the peculiar regions at the periphery. Peripherical cells of spheroids had access to the NPs and could be primarly involved into endocytosis. It needed at least 15 minutes of incubation for the first uptake regions to be separated into irregularly shaped areas. Over the time these areas have rapidly grown directionlessly into the spheroid core (fig. 2 C, D). After that, the nominal regions have shrunk into the single zone with minimal NPs uptake in the center (fig. 2 E). The time and spatial dynamic of AlPc NPs uptake described above could be explained by the heterogeneity of cells in 3D model in temrs of different metabolic processes and phenotypes. Otherwise, the AlPc NPs uptake would have been observed as uniform at the periphery and slightly decreasing towards the spheroid core concentrically.
The numerical estimation of PS uptake in various areas was obtained by recording fluorescence spectra (fig. 3). Before starting the analysis it is worth introducing the equivalent diameter, needed in the presence of a non-perfect sphericity, and defined as the diameter of a circle with 150 μm, corresponding to the average spheroid’s size and having the same area as the spheroid section being imaged. Thus, the total fluorescent signal from the single area was digitized and divided into the auto- and AlPc NPs fluorescent contributions (fig. 3 A, B). Autofluorescence signal was in the spectral range of 430–630 nm, excited by the λex ~ 488 nm. AlPc NPs fluorescence maximum was about 670 nm, excited by the λex ~ 633 nm
(fig. 3). Analysis of spectra from the concentric regions had shown that the AlPc NPs uptake decreased with the autofluorescence increasing from the periphery to the center of spheroid (fig. 3 C).
At the same time the PS NPs uptake distribution was represented by the spheroid’s sections of 4 projections to simplify visual perception, considering PS NPs fluorescence signal alone (fig. 4). This also demonstrates the maximum of PS uptake in the periphery with local minimum in the center.
DISCUSSION
The penetration ability and phototoxicity of AlPc NPs was tested by the confocal laser scanning microscopy. Endocytosis was assumed to be the kind of uptake of NPs. The NPs penetration into the depth of the spheroid was observed over the first hour. However, under the assumption that the multicellular 3D model is homogeneous, NPs should be able to penetrate deep into the spheroids with a uniform volume distribution, which was not observed. So, likely, the uptake diversity in different spheroid’s areas is due to the heterogeneity of spheroid model containing the cells of different phenotypes. This assumption is confirmed by the variety of the phototoxicity effect in the different spheroid’s regions, depending on the presense of oxygen.
Therefore, the tumor model oxygenation was estimated indirectly. In this way, this model for investigating the uptake and photoinduced toxicity of AlPc NPs closely resembles in vivo tumors [14–15].
This result could be explained by the difference in the cells metabolic processes. Indeed, previous studies reported that an apoptotic core begins forming in spheroids of approximately 150–200 μm in diameter [16]. Similar to in vivo tumors, multicellular spheroids include hypoxic and apoptotic/ necrotic areas, developing as a consequence of the formation of oxygen and nutrient gradients. Remarkably, in spheroids, hypoxia occurs gradually over time, with the increase of the spheroid size [17]. Thus, the AlPc NPs uptake gradient could be explained by nutrient gradients and phenotype differences in the cells of 3D model. The degree of the molecular oxygen avalaibility in the different regions can be estimated by the rate of fluorescence signal decrease during the photodynamic irradiation upon the condition that phototoxicity depends on the presence of molecular oxygen. Phototreatment induces the energy transfer as a consecuence of PS fluorescence parching and also a production of active oxygen forms leading to cell death. The areas with a strong photodynamic effect were identified by a comparison of AlPc NPs fluorescence before and after PDT. Analysing spheroid regions, the residual PS fluorescence signal was observed only in the core, while elsewhere the PS fluorescence was absent (fig. 5). It can be related to the heterogeneous structure of spheroid with the various accessibility of deep layers to irradiation light and with different cells proliferation activity. In particular, this means that molecular oxygen is absent in the cental spheroid area, leading to limited photodynamic effect with partial fluorescence decline. Thus, the oxygen gradient in spheroids could be indirectly estimated analysing PS phototoxicity effects. That is also inherent to in vivo features of tumors such as hypoxia.
Finally, the photoxicity was estimated by analysing the spheroid cells viability using the living and dead cells staining. It was shown in comparison with staining of primary spheroid with green marked living cells (fig. 6 A). This evidence consists in the detection of green marking living cells only in the spheroid’s core after PDT (fig. 6 B). At the same time red marked dead cells constitute the main bulk (fig. 6 B). It should be noted that the distribution of standard dye is homogeneous due the staining of cells viability only.
High PS accumulation and penetration are the most important characteristics responsible for anti-tumor efficiency. These characteristics must be carefully considered for novel PS screening. Furthermore, the main factor of incomplete tumor eradication is the PS heterogeneous distribution into the tumor. That is why the complex spatial and temporal distribution processes in tissues are especially important. The spheroid models allow simulating the penetration and intratumor transport of photosensitizer nanoparticles. Nowadays numerous nanoparticles have been studied for efficient and targeted PS delivery. The negative feature of some nanocarriers is the limited penetration, but presumably the size of nanoparticles matters significantly [18–19]. Summarazing the research results, the AlPc NPs are the promising PS with high phototoxicity and, more importantly, AlPc NPs are the probe for the inderect analysis of oxygen distribution, phenotype and metabolic cell processes. At the same time by the AlPc fluorescence estimation it was observed that the 3D multicellular model possesses primary in vivo features of tumors such as intercellular interaction, heterogeneity, hypoxia, oxygen and nutrient gradients. Thus, we suggest that in vitro spheroid model is a good predictive platform for studying the nanosized drugs, including the PS, prior to the animal models.
CONCLUSION
Our investigation clearly demonstrated an advantage of using AlPc nanoparticles as photosensitizer and multifunctional fluorescence probe. AlPc NPs have the sufficient capacity to accumulate, diffuse and penetrate into the spheroids. Microscopy techniques demonstrated that besides sufficient accumulation, AlPc NPs have the dynamic photoactivity depending on the bioenvironment. Particularly, AlPc NPs were used to evaluate the heterogeneity and to indirectly estimate the oxygen concentration, phenotype and metabolic cell processes. These are the most important parameters for the specific local nanophototheranostics. Received results should be useful for the other sighting studies of cell models, for example using the co-culture spheroids, which are taken into the account the immune response [20–24].